Fellows Corner
Formicary, [1] or ant-nest corrosion [2] – based upon the similarity of substrate damage with an ant’s nest (Figure 1) – dates back about fifty years. [3,4]. Formicary corrosion is a rapid acting process involving the conjoint presence of oxygen (air), moisture and a weak organic acid. It may be regarded as a “hidden” phenomenon because, (a) it is unknown to those who have not experienced it first-hand, and (b) it is challenging to identify, because the surface pin hole leak sites are often so fine that they are not visible to the unaided eye (Figure 2). Generally considered unique to copper, this insidious form of internal corrosion (Figure 1) comprises of micro-pitting networks (akin to tunnels) [5,6] that can fully penetrate small bore tubing in weeks, not years. Pin holes that occur on the outer or inner diameter surfaces are commonly surrounded by zones of discoloured copper, ranging from dull gray/black to red/brown or purple hues (Figure 2). Refrigeration-grade copper tubing, [7] used throughout the HVAC industry, was ravaged for decades (Figure 3) and 10% of all premature failures were attributed to formicary corrosion.[8]
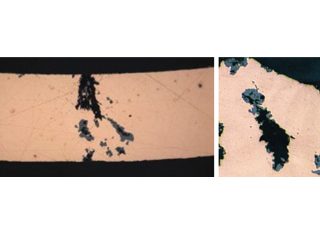
Figure 3: Formicary corrosion common to all HVAC coil manufacturers.
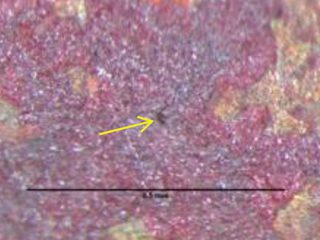
red/brown/purple hues on copper surface.
Figure 2: Tiny pin hole leak location.
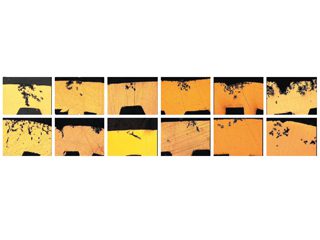
Photomicrographs from different manufacturers; depth of polishing affects the visible spread of damage shown
[9] As-polished.
Copper used in other applications, including heat pumps, dehumidifiers, air coolers, heat exchangers, freezers, and chiller units, can experience formicary corrosion. Identical penetrating attack observed under coatings, lagging, sealant contact, and insulated copper piping, is typically the result of wet conditions and compounds that hydolyse to form carboxylic acids, or other chemical sources, including chlorinated organic compounds (trichlorethane, trichloethylene, etc.), and hydrolysis products from the decomposition of esters, aldehydes and alcohols (carbonyls).
Mechanism of formicary corrosion
Extensive research by the Japanese Copper Development Association [10,11] showed that formicary corrosion occurs when certain residual organic compounds degrade in the simultaneous presence of air and moisture, to produce carboxylic acids, such as formic, acetic, propionic and buteric acids. This finding was pertinent to the HVAC industry (Figure3), where synthetic lubricating oils used for forming coils, and degreasers/detergent cleaners, contained such compounds.
The general mechanism involves a micro anode, where dissolved copper ions combine with carboxylic acids (HCOOO-) to form an unstable cuprous complex Cu(CHOO), which is oxidised to form cupric formate, acetate, etc., 2Cu(CHOO)2 (cuprous complex) and cuprous oxide (Cu2O).
Microcracks with localised intergranular attack, caused by a wedging effect from the volume expansion in forming the cuprous complex and cuprous oxide [6,12] initiate at weaknesses along the corrosion pit wall, exposing more surfaces of copper to perpetuate the advancing corrosion process.
Recognising formicary corrosion
Once the locations of the fine pinholes are found – by water immersion pressure testing, macroscopy, or both – optical microscopy will confirm if the images display the unique metallography of the formicary tunnels (Figures 1, 3), which are significantly different from the smooth hemispherical contours associated with general pitting corrosion of copper (Figure 4).
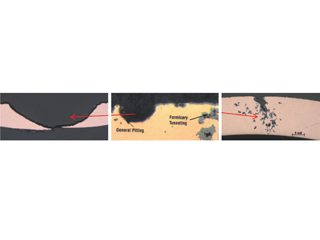
General pitting by halides (chlorides, fluorides). Comparison from coil manufacturer’s report[9]. Subsurface tunnels of formicary corrosion.
Figure 4: Contrasting the two most cited common forms of coil corrosion.
As industries have become more familiar with the causes of formicary corrosion, the frequency of leaks has fallen. Research [13]1 sponsored by the Air Refrigeration Technology Institute (ARTI), to develop a reproducible screening method to determine the mechanisms and effects of corrosion,[14] resulted in a hydrolytic stability test, which uses ion chromatography to ensure that lubricant drawing oils and finning lubricants are free of carboxlic species. A separate pre-screening hexane rinse of copper tubing, provides evidence of residues with carboxylic species identified using Fourier Transform Infrared Spectroscopy (FTIR). Several HVAC equipment manufacturers have made material and design changes, including, tin-plated copper hair pins and tubing less prone to attack, ductless AC units with moisture filters, and all-aluminium tubing and fin construction.
Currently, indoor coil leak failures by formicary corrosion have been attributed to energy- efficient buildings with decreased ventilation that promulgate higher concentration levels of carboxylic acids from building materials, woods, adhesives, disinfectants, cleaning solvents, vinegar seasonings, liquid smoke, cosmetics, etc.
Selected examples of formicary corrosion leakages presented herein, include, HVAC coils with pin holes on the outer (OD) or inner (ID) diameter surfaces (Figures 5, 6), and a heat exchanger from a fabrication shop (Figure 7).
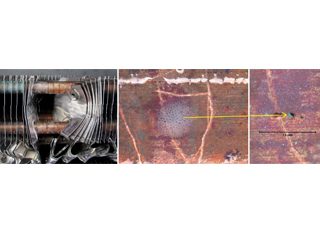
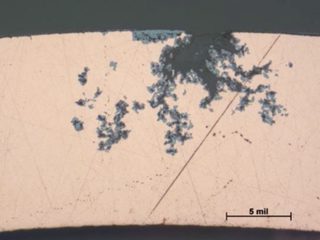
OD-initiated formicary intrusions in defect-free copper.
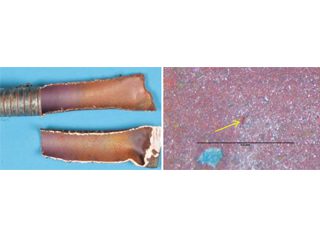
ID of split tube with purple/red-brown surface film. pin hole in purple/red-brown surface film.
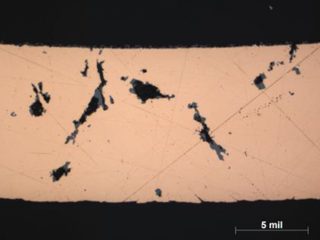
ID-initiated formicary intrusions in defect-free copper.
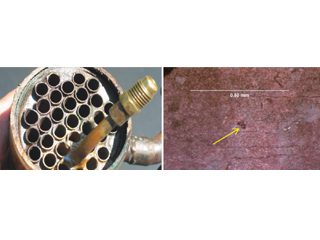
Open end of heat exchanger. Pin hole in purple/red-brown surface film on ID of tube.
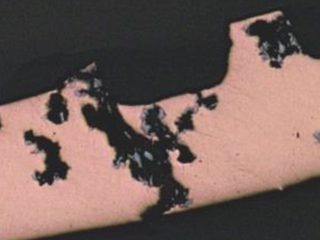
ID-initiated coarse formicary tunnel in defect-free copper.
Pin holes in copper are not always formicary corrosion
Some examples of formicary corrosion have been presented in the previous paragraphs, with brief comments about how it can be assessed and generally avoided by using screening tests on the copper tubing and of the contact environment. Presented In the following paragraphs are some examples where pin holes were wrongly ascribed to formicary corrosion.
• Failed HVAC installation – pin holes found following about 2 to 3 years service were claimed by the coil supplier[15] to display the “worst case of formicary corrosion ever seen”. They were incorrect. The observed damage (Figure 8), and the optical photomicrographs (Figure 9), show no micro-pitting networks – the chacteristic feature for formicary corrosion.
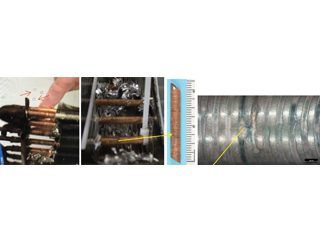
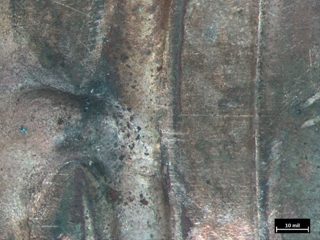
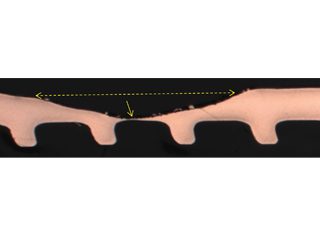
The leak locations along the copper tubing appear to be associated with the design and assembly of the ceiling-mounted units. The repeating geometric pattern of hemispherical- shaped wastage zones close to, or in direct contact with, the aluminium fins, (Figure 9) suggests a modified form of Rosette corrosion,[16] combined with crevice corrosion,[17] and galvanic attack. [18] The additional pitting in the vicinity of the through-wall leaks (Figure 8) is indicative of some form of synergy between these processes.
Rosette corrosion is a somewhat recently recognised corrosion phenomenon,[19] which was encountered in copper hot water cylinders fitted with aluminium anodes. The anodes were installed to prevent type 1 pitting corrosion and was very successfully applied for over 30 years.[20] However, corrosion failures were occurring at the bottom of these cylinders where the water was cooler. This phenomenon was attributed to the interaction of the copper-aluminium galvanic couple [21] and certain impurities in the water that generated reducing species that led to the corrosion at the bottom of the cylinders. This form of corrosion has been essentially eradicated in the United Kingdom, when specifications disallowed the use of aluminium protector rods in 2002.[22] The removal of the aluminium anode and a redesign of the hot water cylinders eliminated the cold bottom thus promoting the growth of a semi-protective corrosion layer.
• Formicary corrosion by contact with sealant – accounting for a blue discoloration surrounding a usually white-colored acoustic sealant (Figure 10), a recent publication wrongly implied that tube failure by formicary corrosion and environmentally assisted cracking (EAC) from such contact was probable. [23] Long-term simulation testing with high relative humidities and temperatures showed this was most unlikely.[24] Without a sustained presence of air and moisture, through-wall penetration by formicary corrosion – a rapid process, as noted earlier – is not expected. Mechanical laps from tube-forming (Figure 10) were wrongly interpreted as EAC with formicary corrosion. This matter was addressed nine years ago, [25] the blue discoloration was nothing more than an aesthetic issue.
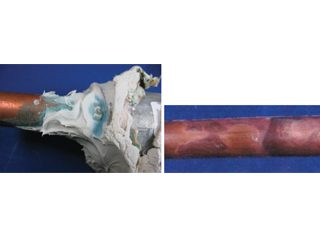
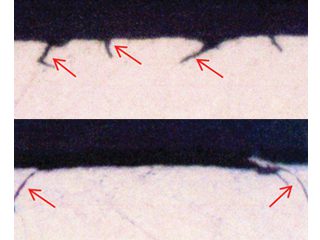
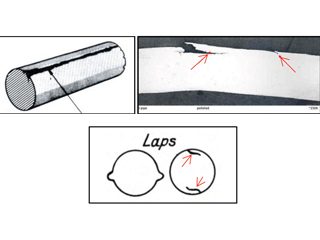
Discussion and conclusions
Formicary corrosion is regarded as a subsurface network of microscopic-corroded tunnels that are considerably larger than the tiny pin holes they connect to, [27] which presents a challenge to those who are aware of the “usual” localised phenomenon of pitting corrosion,which ranked fourth from seven common sources of copper tube corrosion based on over 1,500 investigations over 25 years in domestic water systems. [28] This source noted that all can be mitigated cost-effectively provided that, water quality is maintained, copper tube systems are properly designed and installed, and systems are operated within design parameters.
Formicary corrosion may be active if:
• fluids leak in weeks or months.
• surface zones of discoloured copper (dull gray/black to red/brown or purple hues) are evident.
• copper exposed to air (oxygen), moisture and a weak organic acid, e.g., carboxylic acids, (formic, acetic, propionic, buteric).
• copper exposed to other chemical sources, including chlorinated organic compounds and hydrolysis products from decomposition of esters, aldehydes and alcohols (carbonyls).
• wet conditions persist under coatings, lagging, sealant contact, and insulated piping with compouds that hydrolyse to form carboxylic
acids.
Success in dealing with formicary corrosion, like many other forms of corrosion, is dependent on knowing what it is; knowing how to recognise it; knowing what causes it; and focusing on control, rather than elimination – the realistic goal. [30]
Acknowledgements
The author kindly acknowledges the contributions of Mr. Fred Sherman, Sr. Materials Analyst, for his meticulous contributions to the metallography and laboratory testing, and Mr. Brad Krantz, VP of Laboratory Services, for support and access to Corrosion Testing Laboratories, Newark DE.
References
1.
“For-mi-car-y”: from Medieval Latin, Formica ‘ant’, defined as a nest of ants or an anthill, Oxford Dictionary.
2.
S. Yamauchi, K. Nagata, S. Sato, M. Shimono, J. Japanese Copper & Brass Research Assocn., 22, p.132, (1981).
3.
J.O. Edwards, R.J. Hamilton, J.B. Gilmore, Materials Performance, NACE International, 16, 9, p.18, (1977).
4.
J. M. Keyes, International Copper Research Association Symposium, Belgium, June (1965).
5.
P. Elliott, R.A. Corbett, “Ant Nest Corrosion – Exploring the Labyrinth”, Corrosion Reviews, 19, No. 1, p.1-14, (2001).
6.
R.A. Corbett, P. Elliott, “Digging the Tunnels”, Corrosion Reviews, 20, No. 2, p.51-66, (2002).
7.
DHP copper, alloy C122, UNS C12200, 99.90% copper, ASTM B280.
8.
Go Isobe et al, NACE Corrosion Asia, Paper 105, Singapore, September (1992).
9.
Carrier Corporation, Industry Research Reports “Indoor Coil Corrosion” (2007 to 2011).
10.
T. Notoya, T. Hamamoto, K. Kawano, Corrosion Engineering (Japan), 367, 2, p.1, (1988).
11.
T. Notoya, “Localized “Ant Nest” Corrosion of Copper Tubing and Preventive Measures”,Materials Performance, NACE International, 32, 5, p.53, (1993).
12
D. M. Bastidas, I. Caynela, J.M. Bastidas, CENIM – National Centre for Metallurgical Research, CSIC, Avda, Madrid, Spain, “Ant-nest corrosion of copper tubing in air-conditioning units”, Revista de Metalurgia, 42 (5) September/October, p.367-381, (2006).
13.
Air Refrigeration Technology Institute (ARTI), Report, 21-CR Research Project 611-50055, R. A. Corbett, Corrosion Testing Laboratory, Newark, DE, with input from P. Elliott and T. Notoya, (2003).
14.
R.A. Corbett, “The Development of a Reproducible Screening Method to Determine the Mechanisms and Effects of Organic Acids and other Contaminants on Corrosion of Aluminium-finned copper tube Heat Exchanger Coils, Corrosion 2004, Paper 04321, NACE International,
New Orleans, LA, (2004).
15. Private communication.
16.
Rosette corrosion – the premature failure of copper hot-water cylinders fitted with aluminium rods to prevent Type 1 copper pitting: R.J. Oliphant, Journal of Chartered Institute of Water & Environmental Management, UK, vol 14, p. 207, July (2007). R.J. Oliphant, Causes of Copper Corrosion in Plumbing Systems, Foundation for Water Research Review, FR/R0007, May (2003).
17.
Crevice corrosion – localised corrosion of a metal or alloy surface at, or immediately adjacent to, an area shielded from full exposure to the environment because of close proximity of the metal or alloy to the surface of another material or an adjacent surface of the same metal or alloy. NACE/ASTM G193-12d, Standard Terminology & Acronyms Relating to Corrosion, (2010).
18.
Galvanic corrosion – accelerated metal corrosion because of electrical contact with a more noble metal or nonmetallic conductor in a corrosive environment. NACE/ASTM G193-12d, Standard Terminology & Acronyms Relating to Corrosion, (2010).
19.
R. Francis, Corrosion of Copper and its Alloys: A Practical Guide for Engineers, NACE International, (2010).
20.
R.J. Oliphant, Journal of Chartered Institute of Water & Environmental Management, UK, vol 14,p. 207, July (2007).
21. V.F. Lacey, British Corrosion Jnl., 7, p.36, (1972).
22.
P. Munn, Causes of Copper Corrosion in Plumbing Systems,3rdEdition, Report FR/R0007, Foundation for Water Research September, (2017).
23.
K. Steiner, “Formicary Corrosion and EAC of Copper Tubes in Contact with Building Sealant”, AMPP Annual Conference, Paper No.17846, AMPP, San Antonio, TX, (2022).27.
24.
P. Elliott, “Blue-Stained Sealants Do Not Imply Catastrophic Corrosion of Medical-Grade Copper Piping”, Materials Performance, NACE International, 52, 6, p.57, (2013).
25. ibid.
26.
Laps sourced from: ASM International, Volume No. 11, Failure Analysis & Prevention, Failures Related to Metalworking, p.82, (2002); Rollason E.C., Metallurgy for Engineers, 4th Ed., Edward Arnold, p. 62, (1973).
27. A.H. Brothers, Mainstream Engineering, Rockledge, Fl – internet search.
28.
J. R. Myers and A. Cohen, “Copper-Tube Corrosion in Domestic-Water Systems”, HPA Engineering: Supplement, June, (2005).
29.
P. Elliott, “Fight Formicary Corrosion”, Chemical Processing, p. 37, November (2005).
Peter Elliott
Full section perforation via formicary “tunnels”. 150X Unique “ant”. 500X
Figure 1: Through-wall formicary corrosion and detail of local “ant”.
Removing aluminium fins to expose tiny holes. Soapy bubbles locate miniscule holes by immersion testing with nitrogen.
Figure 5: OD attack in HVAC coil promoted by cleaning/disinfecting wipes containing ethanol, propanol, and a propionate (a carboxylic specie).
OD-initiated formicary intrusions in defect-free copper.
ID of split tube with purple/red-brown surface film. pin hole in purple/red-brown surface film.
Figure 6: ID attack in HVAC tubing by detergent or degreaser contaminated with carboxylic specie. The tube was drawn using lube oil free from carboxylic acids.
ID-initiated formicary intrusions in defect-free copper.
Open end of heat exchanger. Pin hole in purple/red-brown surface film on ID of tube.
Figure 7: ID attack in enhanced copper tubing in a heat exchanger bundle from a fabrication shop. Surface products contained carboxylic (formate) species.
ID-initiated coarse formicary tunnel in defect-free copper.
From left to right. Bubbles show leaks. Aluminium fins stripped to access copper sample. Macro of pinholes on the OD surface.
Close up of OD pinhole leak and surrounding small pits.
Figure 8: Pin hole and other localised pits in one of many HVAC indoor coils.
Detail of OD leak location. As polished. 80X
Figure 9: Through-wall leak location coincides with a repeating geometric pattern of smooth hemispherical pits aligned with the aluminium fin locations.
Blue-stained white sealant; 18 months in service. Superficial etch stains on copper with sealant removed.
Shallow surface laps under sealant. As polished. 500x. Shallow surface laps remote from sealant. 500x
OD – longitudinal surface lap.Photomicrograph of surface laps.Schematics of OD and ID laps.
Figure 10, Blue-stained sealant on copper tubing in hospital installation and shallow surface laps [26] misinterpreted as EACs and the initiation of formicary corrosion.
Fellows Corner
This series of articles is intended to highlight industry-wide engineering experiences, guidance, and focused advice to practising technologists. The series is written by ICorr Fellows who have made significant contributions to the field of corrosion management. This issue features articles on the use of existing pipelines for the transport of hydrogen, by Frank Cheng, Dept. of Mechanical & Manufacturing Engineering, University of Calgary, Canada, and on an interesting aspect of copper corrosion, by Peter Elliott, Corrosion & Materials Consultancy, Inc., Las Vegas, USA.
Suitability of existing pipelines for hydrogen service
It is believed that hydrogen will play a critical role in energy transition, and achievement of the 2050 net-zero emission goal. Hydrogen delivery is integral to the entire value chain of hydrogen economy. Compared with other transportation modes, such as tankers, pipelines provide an economic and efficient means to transport gaseous hydrogen with a high transportation capacity over long distances [1]. Particularly, repurposing existing natural gas pipelines is “a low-cost option for delivering large volumes of hydrogen” [2], contributing to accelerated realisation of a full-scale hydrogen economy, while saving high initial capital costs of constructing new hydrogen-dedicated pipelines.
However, hydrogen embrittlement (HE) can occur on pipelines transporting hydrogen in either blended or pure form, compromising the structural integrity to cause pipeline failures. Compared with newly constructed pipelines, the existing pipelines, after a long time of service in the field, include additional challenges to address in terms of the HE occurrence when converted for hydrogen service.
What is hydrogen embrittlement?
Hydrogen embrittlement is a general term describing the degradation of a material’s (mainly metal) properties and structural integrity, due to hydrogen-metal interactions. HE is a concept serving as an ‘umbrella’ under which many different modes of metallic degradation induced by hydrogen are referred to.
The first mode of HE phenomenon is hydrogen-induced cracking (HIC), which is also regarded as the most dangerous mode of HE-associated materials failure. The HIC occurs when the hydrogen atom concentration at a local site exceeds a threshold value under a given stress condition. Generally, the threshold H atom concentration required to initiate cracks in steels decreases with an increased stress. Many mechanisms or theories have been proposed to explain the HIC occurrence, such as hydrogen-enhanced localised plasticity (HELP), hydrogen-enhanced decohesion (HEDE), adsorption- induced dislocation emission (AIDE), and hydrogen-assisted microvoid coalescence (HAMC) [3].
Presently the dominant mechanisms for HIC of steels are the HEDE and HELP [4]. According to the HEDE mechanism, H atoms can weaken bonds between iron atoms in steels and when the external stress exceeds the atomic cohesive strength of Fe in the presence of H atoms, microcracks can be initiated. The HELP mechanism proposes that H atoms accumulate at dislocations to decrease the interfacial elastic energy between mobile dislocations, enhancing the mobility of the dislocations. As a result, the local deformation can occur at a lowered stress, facilitating plastic deformation and cracking occurrence. The HIC can initiate whether on the surface of a metal (i.e., external HIC,) or inside the metal (i.e., internal HIC).
The second mode of HE is hydrogen blistering, where the H atoms entering the metal become recombined to form hydrogen molecules (H2) at the trapping sites, such as voids. The elevated pressure due to accumulation of H2 molecules at a local site can cause formation of a blister (or bubble) on the metal surface. This hydrogen blistering usually occurs on low-strength and ductile metals where the bubbling process does not initiate cracks. Instead, the metals’ experience a remarkable local plastic deformation. If the metals have a high strength and limited ductility, the hydrogen-elevated pressure can cause cracking by the so-called hydrogen internal pressure (HIP) mechanism.
The most common mode of HE phenomenon is degradation of mechanical properties of the affected metals, which is usually shown as a reduction in fracture toughness and ductility. The direct evidence of this mode is the decrease in fracture elongation in the stress-strain curve measured on hydrogen- charged metals, compared with the tensile behaviour of hydrogen-free metals. Generally, a limited amount of hydrogen atoms may not be sufficient to initiate cracks, but can cause a reduction in ductility of the metals.
Uniqueness of pipeline hydrogen embrittlement in high-pressure gaseous environments
HE can occur on pipelines in high-pressure gaseous hydrogen environments. The entire HIC processes include six steps, i.e., generation of H atoms, adsorption of the H atoms on the steel surface, absorption of the H atoms by permeating into the steel, diffusion of H atoms in the crystalline lattice, trapping (accumulation) of H atoms at local sites, and the crack initiation, as schematically shown in Figure 1. The first three steps, i.e., H atom generation, adsorption, and absorption, depend heavily on the environment, whereas the other steps of diffusion, trapping and cracking, mainly rely on the metallurgical factors.
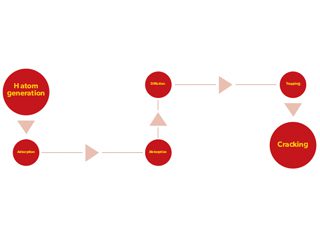
Figure 1. Entire processes for HIC occurrence on metals (steels).
Previous work demonstrated that H atoms can generate and become adsorbed on steel surfaces through a so-called dissociative adsorption mechanism in high-pressure gaseous environments [5]. The Gibbs free energy changes for production of H atoms from gaseous H2 molecules are negative, indicating a thermodynamic feasibility, under pipeline operating conditions with typical temperatures, pressures and H2 gas/natural gas blending ratios. Moreover, the generated H atoms can adsorb stably at On-Top (OT) and 2-fold (2F) Cross-Bridge sites of the Fe (100) crystalline plane, while the hydrogen adsorption at 2F sites is more stable due to a higher electron density and a stronger electronic hybridisation between Fe and H. Upon entering the steels, the H atoms predominantly stay at tetrahedral void sites due to a low energy path and exothermic feature. It has been noted that a uniform distribution of H atoms at the tetrahedral voids in a crystalline lattice would not cause HE or HIC, if the amount of H atoms is within the limit of H solubility. However, the local solubility can be exceeded if H atoms diffuse towards high stress zones, or become trapped at metallurgical features such as grain boundaries, dislocations, and non-metallic inclusions.
Nowadays, substantial studies have been conducted to investigate HE of metals, including pipeline steels, in aqueous environments, where most investigations focused on H atom generation during steel corrosion or cathodic over-protection. Four major differences exist in H atom generation and permeation in steel between a gaseous environment (“gaseous” hydrogen) and the aqueous environment (“cathodic” hydrogen), as listed in Table 1, making it infeasible to directly use data obtained in aqueous environments for HE investigations on pipelines in high-pressure gaseous environments. First, the amount of “cathodic” hydrogen generating during electrochemical cathodic charging, either potentiostatically or galvanostatically, is always substantial. A significant H atom concentration gradient exists between the outside and inside subsurface of the steel. However, the amount of H atoms generated in a gaseous environment is usually limited. After several months of exposure of a pipe steel to high-pressure (10 MPa) H2 gas, the measured H concentration is less than 1 ppm [6]. Secondly, due to the high concentration gradient of H atoms across the steel surface, the permeation of “cathodic” hydrogen is always one way, entering the steel from the adsorption to absorption state. As a comparison, the “gaseous” H atoms, even entering the steel and becoming absorbed inside, can still leave the absorption site and change to adsorption state [5]. Thus, the permeation of “gaseous” hydrogen is reversible. Third, the electrochemical hydrogen-charging usually generates reproducible results, making the testing method (i.e., the Devanathan-Stachurski cell) a standard method for hydrogen atom permeation tests [7]. Although there has been limited work to measure the H atom permeation in gaseous environments, the obtained data are usually scattered, and sometimes, even conflict each other. Finally, both constant concentration model and constant flux model have been developed to fit the electrochemical cathodic H-charging results to derive the H permeation parameters such as H diffusivity, subsurface H concentration and trapping density [8]. To date, a model applied for “gaseous” hydrogen permeation has not been established. Due to the differences listed, the testing results and modeling methods for aqueous “cathodic” hydrogen testing cannot be used for gaseous hydrogen permeation testing.
‘Gaseous’
hydrogen ‘Cathodic’
hydrogen
Amount generated to adsorb on steel surface Limited Substantial
Permeation pathway Reversible between adsorption and absorption One way from adsorption to absorption
Testing results Scattering, and sometimes, controversial Reproducible
Numerical model to derive hydrogen permeating parameters None Constant concentration model and constant flux model
Table 1. Comparison of the H atom generation and permeation in steels in a gaseous environment and in an aqueous environment.
Additional challenges when repurposing existing pipelines for hydrogen service
After a long time of service in the field, existing pipelines become aged and contain various surface defects such as dents, corrosion, scratches, winkles and microcracks. These defects, if passing the assessment criteria [9], would not be required to be repaired, and the pipelines can continue to operate. However, they serve as effective traps to accumulate H atoms. Particularly, dents, a common type of mechanical damage present on pipelines, is a permanent inward deformation on the pipe body, greatly changing the local stress and strain distributions. As a result, the H atoms, once entering the pipe steels, tend to diffuse towards the dent and accumulating locally. Modeling results showed that there were maximum H atom concentrations at both sides of the dent along the circumferential direction, as shown in Figure 2. The H atom distribution coincided with the distribution of hydrostatic stress on the pipe. Moreover, as the dent depth increases, the H atom concentration increases at the local area.
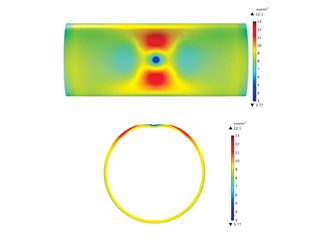
Figure 2. Distributions of the H atom concentration (unit: mol/m3) on an X52 steel pipe segment containing a constrained dent and the cross-sectional view. The dent depth is 3% of pipe outer diameter and the internal pressure is 10 MPa [10].
Different from a dent, a corrosion defect causes metal loss on the pipe wall. Moreover, the corrosion defect is dynamic in nature, and will grow with time in the service environment. It is acknowledged that corrosion is one of the primary mechanisms causing pipeline failures. Generally, the presence of a corrosion defect on the pipe body can decrease the pressure-bearing capability of the pipelines.
Thus, many standards and codes have been developed for corrosion defect assessment. Similar to a dent, if a corrosion defect passes the assessment criteria, the corroded pipelines can continue to operate, without a need to repair the corrosion defect, but a cautious monitoring of the defect growth should be followed. It was confirmed that the H atoms which were originally distributed in the crystalline lattice of a steel would diffuse towards the corrosion defect. Moreover, as the corrosion defect length reduces and the depth increases, the H atom concentration becomes more apparent, as seen in Figure 3.
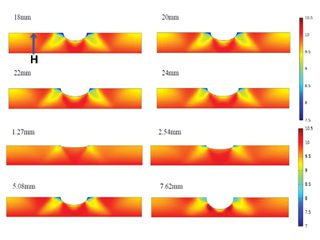
Figure 3. Distributions of the H atom concentration (unit: mol/m3) on an X52 steel pipe segment containing a corrosion defect with varied lengths and depths [10].
A technical assessment programme for the suitability of aged pipelines in hydrogen service
Obviously, a technical programme must be developed to assess the suitability of the existing aged pipelines to transport hydrogen in either pure or blended form. The assessment should consider the synergism of steel metallurgy, stress, and hydrogen, on HE or HIC occurrence. Particularly, the surface defects present on the pipelines should be paid much attention, in addition to the metallurgical features such as grain boundaries, dislocations and non-metallic inclusions, which are effective hydrogen traps. The specific service history of the pipelines which were made of various grades of steel should be considered, and the technical assessment should be conducted case by case. Once successfully developed, the technical assessment programme will be able to: evaluate the possibility of H atoms generation and the amount of H atoms adsorbed on the steel surface under pipeline operating conditions, quantify the accumulated H atom concentration at the surface defects and metallurgical features under given conditions, estimate the threshold H atom concentration at a local defect to initiate cracks under a certain stressing condition, rank the HE susceptibility of the aged pipelines while considering the metallurgical and stress factors, and recommend proper operating conditions (e.g., temperature, pressure and blending ratio) to minimise and eliminate pipeline HE in high-pressure gaseous environments.
References
[1]
A.S. Hawkins, Technological Characterization of Hydrogen Storage and Distribution Technologies, UKSHEC Social Science Working paper no. 21, Policy Studies Institute, London, UK, 2006.
[2]
U.S. Department of Energy, Hydrogen Pipelines, Washington DC, US, 2020.
[3]
Yinghao Sun, Y. Frank Cheng, Hydrogen-induced degradation of high-strength steel pipeline welds: A critical review, Eng. Fail. Anal, 133 (2022) 105985.
[4]
M.B. Djukic, G.M. Bakic, V. Sijacki Zeravcic, A. Sedmak, B. Rajicic, The synergistic action and interplay of hydrogen embrittlement mechanisms in steels and iron: Localized plasticity and decohesion, Eng. Frac. Mech., 216 (2019) 106528–106561.
[5]
Yinghao Sun, Y. Frank Cheng, Thermodynamics of spontaneous dissociation and dissociative adsorption of hydrogen molecules and hydrogen atom adsorption and absorption on steel under pipelining conditions, Int. J. Hydrogen Energy, 46 (2021) 34469-34486.
[6]
G. Golisch, G. Genchev, E. Wanzenberg, J. Mentz, H. Brauer, E. Muth
mann, D. Ratke, Application of line pipe and hot induction bends in hydrogen gas, J. Pipeline Sci. Eng., 2 (2022) 100067.
[7]
M.A.V. Devanathan, Z. Stachurski, The adsorption and diffusion of electrolytic hydrogen in palladium, Proc. Royal Soc., A270 (1962) 90-102.
[8]
Y.F. Cheng, Analysis of electrochemical hydrogen permeation through X-65 pipeline steel and its implications on pipeline stress corrosion cracking, Int. J. Hydrogen Energy, 32 (2007) 1269–1276.
[9]
Guojin Qin, Y. Frank Cheng, A review on defect assessment of pipelines: Principles, numerical solutions, and applications, Int. J. Press. Vessel Pip., 191 (2021) 104329.
[10]
Frank Cheng, Hydrogen transport in aged pipelines II. Technical assessment of the susceptibility to hydrogen embrittlement, AMPP / ASM International Calgary Chapter Luncheon, Calgary, Canada, Oct. 19, 2022.
Frank Cheng